Q: What do you mean when you say you’re “seeing some of the earliest galaxies in the universe?” How does looking into deep space allow you to look back in time?
The simple answer is that light travels and the universe is huge. Light travels very fast – 186,000 miles (300,000 km) per second, but it still has to move across the vast distances of space. Remember that for us to see anything – from the flash of a camera to the glow of a really distant galaxy, we have to wait for its light to strike our eyes.
That camera flash shows in our vision instantaneously because it doesn’t have far to go. But distances in the cosmos are so vast that it takes light a long time to reach us. The light from our closest companion, the Moon, takes about 1.3 seconds to cross the 239,000 miles (390,000 km) between us. So when you look up at the sky, you don’t see the Moon as it currently is. You see it as it appeared 1.3 seconds ago.

This is so 1.3 seconds ago.
Credit: Luc Viatour, Wikimedia Commons
The greater the distances, the greater the time difference. Light from the Sun needs about 500 seconds, or about eight minutes, to reach us from 93,200 miles (150 million km) away. Light from Neptune needs about four hours to cross the solar system.
We refer to these distances by the time it takes light to cross them. So Neptune is four light-hours away, and the Sun is 500 light-seconds away. Light from the next nearest star, however, needs four years to reach us across space. We say that star is four light-years away. The light we see from that star in today’s sky is also four years old. For galaxies, we’re talking millions to billions of light years. So we see the farthest galaxies as they appeared in the early universe, because the light that left them way back then is finally reaching us just now.
Q: What does it mean when you talk about a galaxy’s redshift?
When we’re discussing the Frontier Fields project, we’re talking about something more precisely called “cosmological redshift.” The space light is traveling through is expanding. That means that the light wave gets stretched as it travels, like a spring being pulled into a different shape. This stretching shifts light into longer wavelengths.

Since red light has a longer wavelength than blue light, the light is said to be “redshifted.” Credit: NASA
The farthest galaxies in the universe would have originally emitted visible and ultraviolet light, but since that light has been stretched as it travels, those galaxies appear to us instead in the form of infrared light. Cosmological redshift refers to that change and the measure of that change.
Q: Why do we hear the Frontier Fields galaxies described in terms of redshift and light-years? Which is right?
They tell us different things. Light-years are a measurement of distance defined by the time it takes light to travel in a year. But distance is notoriously difficult to measure in astronomy.
Cosmological redshift is a direct measurement of the expansion of space. Astronomers describe galaxies in terms of their redshift because unlike distance, it’s a clear and definite value that’s relatively easy to measure without many errors.
Astronomers have different models of how the universe works, and they can plug the redshift into those models to get the distance to a galaxy – but the distance will differ depending on which model of the universe they use. The variations in those models include things like the shape of the universe, the rate at which it’s expanding, the amount of normal matter it contains, etc.
Astronomy is about figuring out how the universe works and narrowing down all those models to the best one, and we still have a long way to go. Projects like Frontier Fields will help us rule out those models that don’t fit the incoming data.
Q: Everywhere we look with the Frontier Fields project, galaxies appear to be moving away from us. Does this mean we’re in the center of the universe?
No. It’s evidence that space is expanding. The easiest way to visualize this is to imagine a balloon. If you cover the balloon with dots, and then inflate it, no matter which dot you pick to represent your position, all the other dots will appear to be moving away from it as the balloon expands. Imagine this happening in three dimensions instead of on a flat surface, and you can understand why it looks like other galaxies are rushing away.
Q: So space is expanding and the light from the earliest galaxies has traveled over 13 billion years to reach us. If space is expanding, are those galaxies even farther away now?
Yes. For nearby galaxies, the expansion doesn’t make much of a difference. But for galaxies extremely far away, the distance is significant. That’s because the farther away an object is, the more space there is between us and the object. That in turn means there’s more space to undergo expansion, so the objects appear to be moving away from us much faster. Light from the earliest galaxies may have traveled 13 billion years to reach us, but those galaxies could be around 45 billion light-years distant by now.
Q: Does this mean the galaxies are moving faster than the speed of light?
No. No object can travel through space faster than the speed of light. But the expansion of space itself is not so constrained – in fact, theories of the beginning of the universe visualize the initial expansion of the Big Bang happening with unthinkable speed. But because the speed of light is only so fast, there are galaxies in the distance whose light we cannot yet see. We call this the edge of the visible universe.
Q: What’s out there, past the edge?
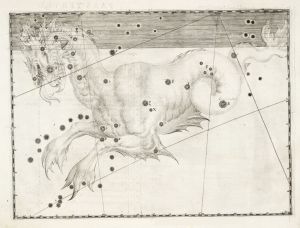
DRAGONS! SPACE DRAGONS! GIANT, COSMIC FIRE-BREATHING SPACE DRA– Ok, fine, probably not. Credit: Uranometria, Wikimedia Commons
We expect more of the same, though this is still an open question that astronomers are researching and theorizing about. We’ve found we tend to see the same distribution of galaxies no matter which direction we look in the universe. If we were somehow transported to a galaxy on what, for Earth, is the edge of the visible universe, the border of the visible universe would move, but the universe would neither change nor look very different to us.
Q: Do you have a question about the Frontier Fields project?
Leave it in comments, and we’ll see if we can answer it.